Research
Merging organometallic chemistry with biotechnology
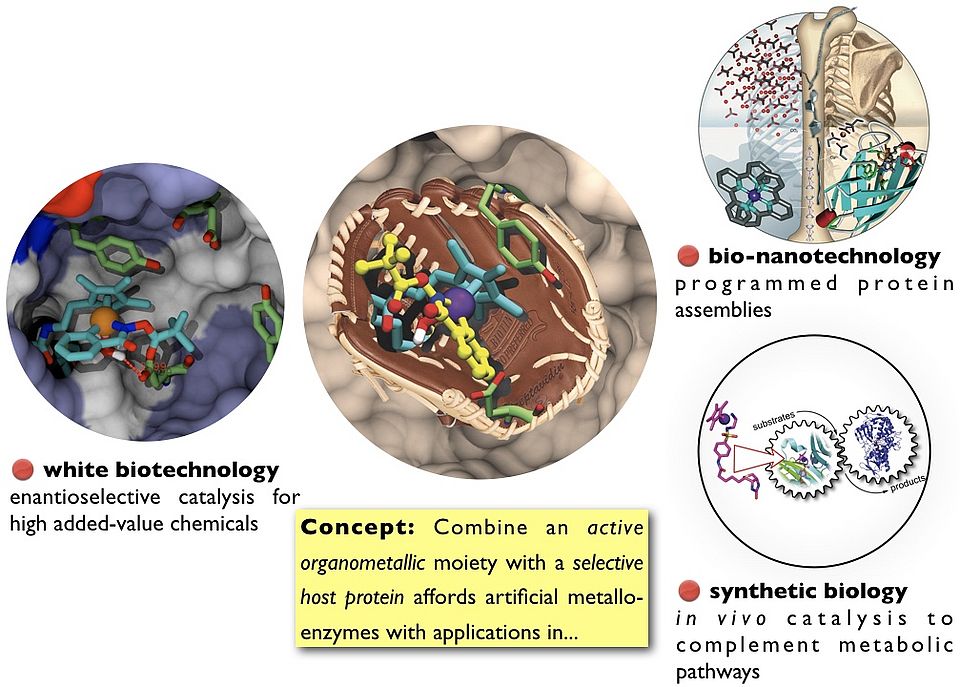
Artificial metalloenzymes
Organometallic- and enzymatic catalysis have evolved independently over the past four decades. In many respects, these approaches can be viewed as complementary. By incorporating an organometallic moiety within a protein host, we create artificial metallo-enzymes, with properties reminiscent both of homogeneous and enzymatic catalysis. The main focus of our research is to exploit such hybrid systems towards various applications.
- Enantioselective catalysis (white biotechnology)
- Synthetic biology (metabolic engineering)
- Bio-nanotechnology
In order to ensure unambiguous localisation of the organometallic moiety within the host protein, we rely on various anchoring strategies.
- Covalent anchoring exploiting carbonic anhydrase as scaffold
- Supramolecular anchoring exploiting the biotin-streptavidin couple
- Dative anchoring upon repurposing proteins with latent facial triad motifs
Basic concept
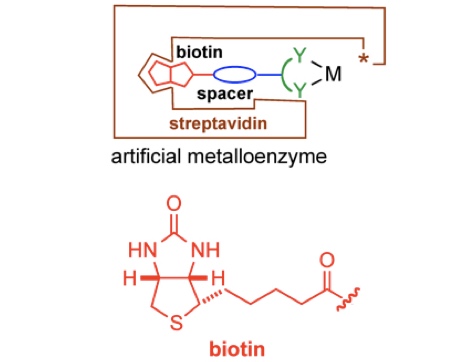
In the spirit of E. Fischer's Lock and Key. The anchor (red; for example biotin) displays a very high affinity for the host protein (brown; for example streptavidin (Ka 1014 M–1)). A catalytically competent organometallic moiety (green), linked via a spacer (blue) to biotin is combined with streptavidin to yield an artificial metalloenzyme. A broadly applicable chemogenetic optimization strategy relies on
i) mutations (*) on streptavidin and
ii) variation of the spacer and the bidentate ligand
This strategy can be applied to any host protein-cofactor couple.
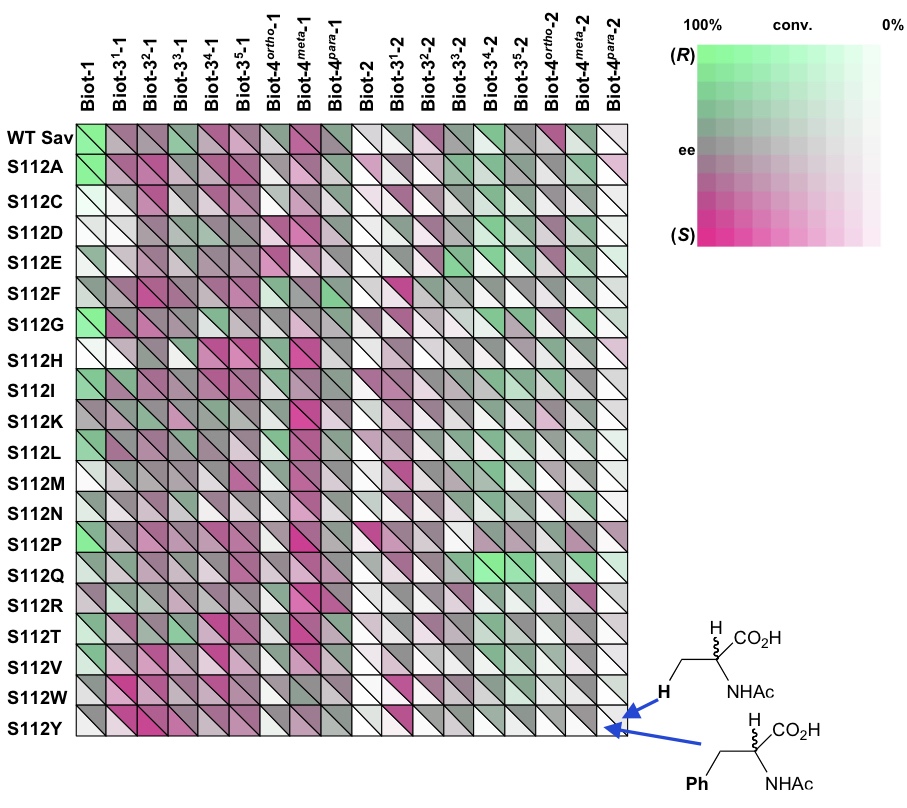
The chemogenetic optimisation strategy combines random mutagenesis (the rows) of the host protein with chemical variation of the anchored cofactor (the columns). After screening various spacers and ligands, a directed evolution protocol allows to screen thousands of mutants with the selected cofactor to identify versatile artificial metalloenzyme.
A Mosaic of applications
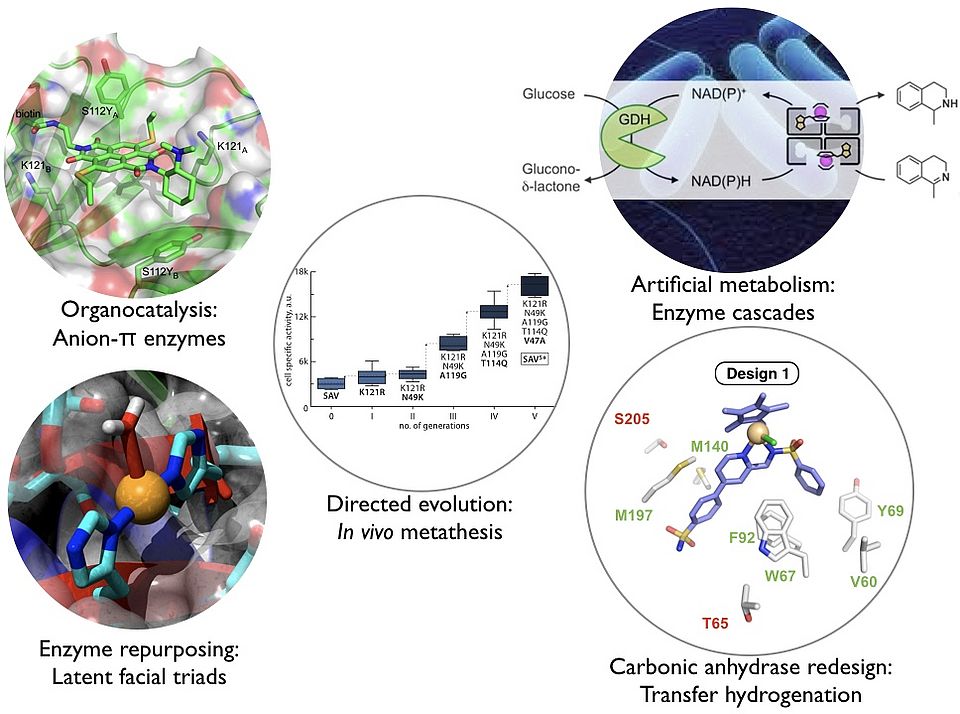
Reactions Implemented
- Hydrogenation
- Transfer hydrogenation
- Ketones, enones, imines, NAD(P)+
- Allylic substitution
- C-H activation
- Suzuki cross-coupling
- Metathesis
- Alcohol oxidation
- Sulfoxidation
- Dihydroxylation
- Peroxidation
- Michael addition
- Enzyme cascades
- DNA recognition
Current
- In vivo catalysis
- Directed evolution
- Alternative metabolism
- Cross-regulation
- Off-equilibrium thermodynamics
- Catalytic drugs
- Biofuels
- Multi-electron processes
- Artificial photosynthesis
Funding
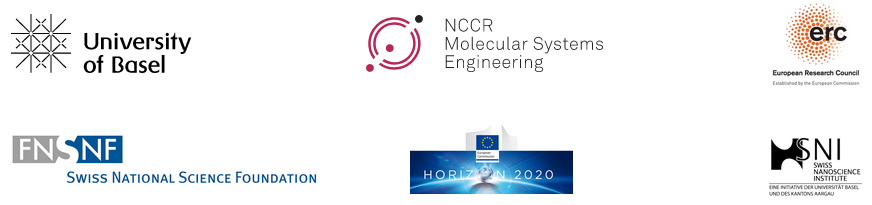
DrEAM — ERC Advanced Grant
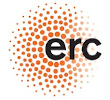
Directed Evolution of Artificial Metalloenzymes for In Vivo Applications
In the past decade, artificial metalloenzymes (AMs) have emerged as an attractive alternative to the more traditional enzymes and homogeneous catalysts. Such hybrid catalysts result from the incorporation of an abiotic metal cofactor within a macromolecule (protein or oligonucleotide). Artificial metalloenzymes combine attractive features of both homogeneous catalysts and enzymes, including the possibility to genetically optimize the catalytic performance of new-to-nature organometallic reactions. Can artificial metalloenzymes become as catalytically efficient as naturally-evolved metalloenzymes, even in complex biological mixtures? Herein, we outline our efforts to address this challenge by localizing and evolving AMs within the periplasm of Escherichia coli.
To achieve this objective, we will exploit AMs based on the biotin-streptavidin technology. Four subprojects have been tailored to address the challenges: i) knock-out deleterious components present in the periplasm; ii) improve the cofactor uptake through the outer-membrane; iii) engineer streptavidin to boost the AM’s performance; and iv) rely both on screening and selection strategies to evolve AMs in vivo. Relying on auxotrophs, we will demonstrate the potential of AMs to complement metabolic pathways. Only E. coli auxotrophs containing an evolved AM capable of producing the vital aminoacid-precursor will survive the stringent selection pressure. We have identified several selectable aminoacid precursors which can be produced by metathesis (indole, precursor of tryptophan), enone reduction (keto valine, precursor of valine) and allylic substitution (prephenate, precursor of tyrosine and phenylalanine). In a Darwinian evolution spirit, we anticipate that applying selection pressure will allow to evolve AMs to unprecedented catalytic performance.
The main deliverable of the DrEAM is an engineered and evolvable E. coli strain capable of performing in vivo reaction cascades combining AMs and natural enzymes.
Publications
Breaking Symmetry: Engineering Single-Chain Dimeric Streptavidin as Host for Artificial Metalloenzymes
S. Wu, Y. Zhou, J.G. Rebelein, M. Kuhn, H. Mallin, J. Zhao, N.V. Igareta, T.R. Ward*
J. Am. Chem. Soc., 2019, 141, 15869. 10.1021/jacs.9b06923
"Close-to-Release": Spontaneous Bioorthogonal Uncaging Resulting from Ring-Closing Metathesis
V. Sabatino, J.G. Rebelein, T.R. Ward
J. Am. Chem. Soc., 2019, 141, 17048. 10.1021/jacs.9b07193
Artificial Metalloenzymes: Challenges and Opportunities
H.J. Davis, T.R. Ward
ACS Cent. Sci., 2019, 5, 1120. 10.1021/acscentsci.9b00397
Chemical Optimization of Whole-Cell Transfer Hydrogenation Using Carbonic Anhydrase as Host Protein
J.G. Rebelein, Y. Cotelle, B. Garabedian, T.R. Ward
ACS Catal., 2019, 9, 4173. 10.1021/acscatal.9b01006
Artificial Metalloenzymes Based on the Biotin–Streptavidin Technology: Enzymatic Cascades and Directed Evolution
A.D. Liang, J. Serrano-Plana, R.L. Peterson, T.R. Ward
Acc. Chem. Res., 2019, 52, 585. 10.1021/acs.accounts.8b00618
Genetic Engineering of an Artificial Metalloenzyme for Transfer Hydrogenation of a Self-Immolative Substrate in Escherichia coli’s Periplasm
J. Zhao, J.G. Rebelein, H. Mallin, C. Trindler, M.M. Pellizzoni, T.R. Ward
J. Am. Chem. Soc., 2018, 140, 13171. 10.1021/jacs.8b07189
Ferritin Encapsulation of Artificial Metalloenzymes: Engineering a Tertiary Coordination Sphere for an Artificial Transfer Hydrogenase
M. Hestericová, T. Heinisch, M. Lenz, T.R. Ward
Dalton Trans., 2018, 47, 10837. 10.1039/c8dt02224k
A Cell-Penetrating Artificial Metalloenzyme Regulates a Gene Switch in a Designer Mammalian Cell
Y. Okamoto, R. Kojima, F. Schwizer, E. Bartolami, T. Heinisch, S.Matile, M. Fussenegger, T.R. Ward
Nat. Commun., 2018, 9, 1943. 10.1038/s41467-018-04440-0
An Artificial Metalloenzyme for Carbene Transfer Based on a Biotinylated Dirhodium Anchored within Streptavidin
J. Zhao, D.G. Bachmann, M. Lenz, D.G. Gillingham, T.R. Ward
Catal. Sci. Technol., 2018, 8, 2294. 10.1039/c8cy00646f
Photo‐Driven Hydrogen Evolution by an Artificial Hydrogenase Utilizing the Biotin‐Streptavidin Technology
S.G. Keller, B. Probst, T. Heinisch, R. Alberto, Roger, T.R. Ward
Helv. Chim. Acta, 2018, 101, e1800036. 10.1002/hlca.201800036
E. coli surface display of streptavidin for directed evolution of an allylic deallylase
T. Heinisch, F. Schwizer, B. Garabedian, E. Csibra, M. Jeschek, J. Vallapurackal, V.B. Pinheiro, P. Marlière, S. Panke, T.R. Ward
Chem. Sci., 2018, 9, 5383. 10.1039/c8sc00484f
Streptavidin‐Enzyme Linked Aggregates for the One‐Step Assembly and Purification of Enzyme Cascades
H. Mallin, T.R. Ward
ChemCatChem, 2018, 10, 2810. 10.1002/cctc.201800162
In Vivo Catalyzed New-to-Nature Reactions
J.G. Rebelein, T.R. Ward
Curr. Opin. Biotechnol., 2018, 53, 106. 10.1016/j.copbio.2017.12.008
Directed Evolution of an Artificial Imine Reductase
M. Hestericová, T. Heinisch, L. Alonso-Cotchico, J-D. Maréchal, P. Vidossich, T.R. Ward
Angew. Chem. Int. Ed., 2018, 57, 1863. 10.1002/anie.201711016
Streptavidin as a Scaffold for Light-Induced Long-Lived Charge Separation
S.G. Keller, A. Pannwitz, H. Mallin, O. Wenger, T.R. Ward
Chem. Eur. J., 2017, 23, 18019. 10.1002/chem.201703885
Cross-Regulation of an Artificial Metalloenzyme
Y. Okamoto, T.R. Ward
Angew. Chem. Int. Ed., 2017, 56, 10156. 10.1002/anie.201702181